By Mary-Elizabeth Jobson
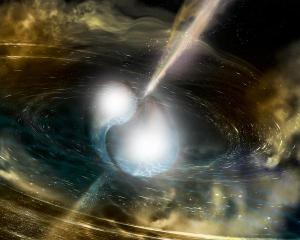
An artist’s conception of two neutron stars colliding to produce powerful jets of energy and gravitational waves. Credit: NSF/LIGO/Sonoma State University/A. Simonnet
Astrophysicists have succeeded in shedding light on previously hidden events that occur deep within the heart of merging neutron stars. Their approach combines precise visualization from modern radio telescopes with extremely sensitive systems that detect faint gravitational waves.
Researchers already received a Nobel Prize for spotting the gravitational signals streaming from an even more cataclysmic collision, between two black holes. But these triumphs are just the beginning for the emerging field, according to scientists who spoke on Feb. 16 at the American Association for the Advancement of Science (AAAS) annual meeting. Observations of these rare phenomena will become commonplace in the next decade as detection technology advances, the speakers said.
Gravitational-wave astronomy “is no longer a novelty,” said David Shoemaker of the Kavli Institute for Astrophysics and Space Research at the Massachusetts Institute of Technology, one of the field’s leaders. The next generation of detectors “will allow people to dream of things that are bigger and farther away,” he said.
Gravitational waves were first proposed by Albert Einstein in 1916 as part of his general theory of relativity. Small distortions in the fabric of space should travel at the speed of light through the universe, Einstein said, arising from energetic events that violently disturb large masses. Modern astronomers have suspected for decades that their best chance to spot these waves directly was to pinpoint a sudden merger between two black holes or two neutron stars—the super-dense remnants of the explosive deaths of massive stars.
These objects stretch the imagination: a single teaspoon of neutron-star matter is as massive as 200 battleships, said Kunal Mooley of the National Radio Astronomy Observatory (NRAO) in Charlottesville, Virginia, and the California Institute of Technology. When a pair of these objects becomes entwined, they whirl ever more tightly and then crash into each other in spectacular fashion, creating a new black hole.
The gravitational waves streaming from such collisions are nearly imperceptible on Earth as they pass, distorting the length of a meter stick by a fraction of the width of a proton. To detect such small signals, researchers use the Laser Interferometer Gravitational-Wave Observatory (LIGO), with two facilities in Washington and Louisiana, along with the Virgo interferometer in Italy.
These interferometers work by bouncing laser light between mirrors at the ends of two 4-kilometer-long arms arranged in an L-shape. (The Virgo arms are 3 kilometers long.) If gravitational waves pass through the labs, the distortion in space stretches and squeezes the lengths of the arms relative to each other. This creates unique signals in the combined light patterns from the two arms, which computers can detect instantly.
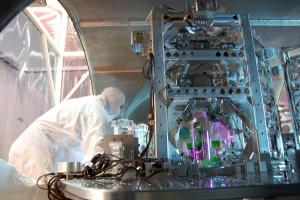
A laser scientist works on a mirror system in one of LIGO's 4-kilometer-long tunnels. Credit: LIGO Laboratory
The announcement of those gravitational waves set off a frantic cascade of action worldwide as astronomers ran to their telescopes to try to pinpoint the source. The merger, they found, happened in a galaxy about 130 million light-years away. Sixteen days later, astronomers spotted the first radio signals from the collision with NRAO’s Very Large Array of radio telescopes in New Mexico.
The team then added data from radio telescopes spanning the U.S. to study these signals in high resolution, allowing them to visualize extremely fine details of the merger. The radio waves unveiled a cloud of neutron-rich matter surrounding massive jets of energy, which burst through the cloud with astonishing speed and power.
By pinpointing the emerging jet, “we solved a mystery that has been haunting astronomers for three decades,” Mooley said. Previously, theorists thought the merger's dense cocoon might “choke” the jet, preventing it from escaping.
On Feb. 14, LIGO received $34 million combined from the National Science Foundation, UK Research and Innovation, and the Australian Research Council to fund Advanced LIGO Plus, to make the interferometers even more sensitive. With these and other planned improvements, detecting gravitational waves will become a daily occurrence, Shoemaker said.
The upgrades promise to reveal other exotic phenomena, the speakers said, such as single exploding stars, rapidly spinning irregular neutron stars, and lingering gravitational waves from the Big Bang itself. In the words of Dario Carbone of Texas Tech University, the landmark neutron-star research from 2017 “is only page one of this book.”
Mary-Elizabeth Jobson is a senior at the University of Florida majoring in microbiology with a focus on astrobiology. Contact her at maryeljobson@gmail.com or view her website at https://mjobson.weebly.com/